Written by Clio Michel, researcher at the Bjerknes Centre and the Geophysical Institute at the University of Bergen
El Niño Southern Oscillation influences the weather over the whole globe. It is therefore of crucial importance to know how it will change in the future. Large ensembles of climate simulations help in determining the accuracy of eventual changes.
Air motions in the atmosphere are largely of chaotic nature which make them very variable and difficult to predict after one week (Washington 2000). To reproduce this natural variability of the atmosphere (also called internal variability), scientists have created large ensembles of model simulations (Deser et al. 2012).
A type of large ensemble is based on the famous “butterfly effect”. The fact that the flapping of the wings of a butterfly, in India for example, can affect the weather in Norway means that very slightly different initial conditions of the atmosphere (at time 0) lead to very large differences at a later time (Lorenz 1963). Therefore, scientists have created large ensembles of simulations by varying the initial conditions given to the models to improve the prediction of both weather and climate and assess the prediction uncertainty.
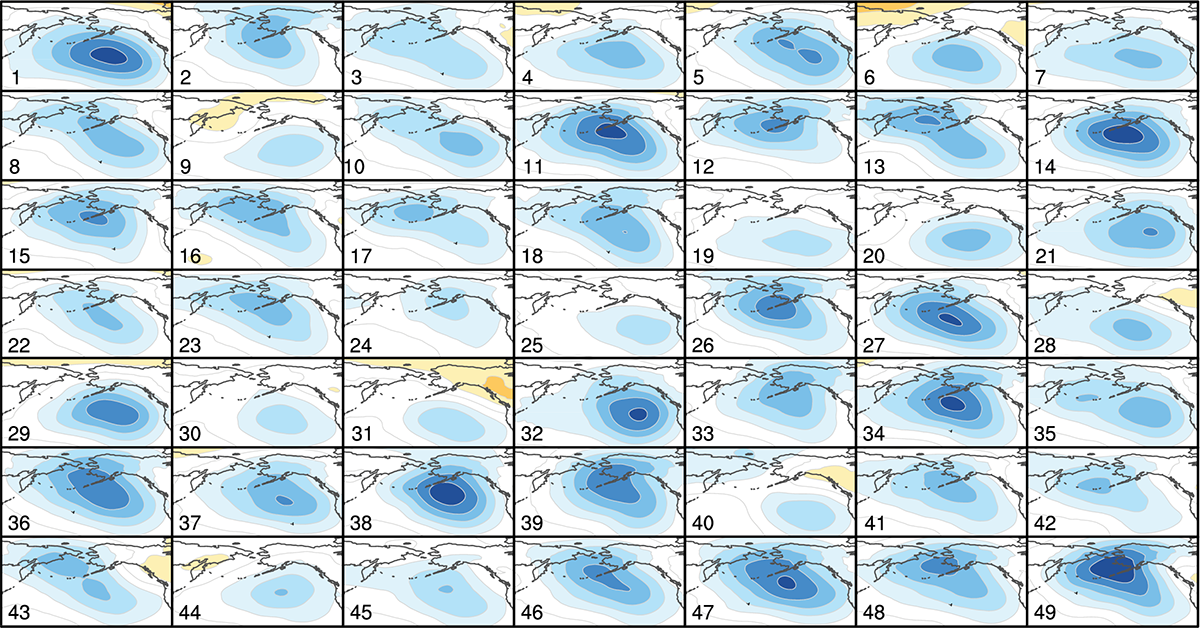
In a recent study, we took advantage of a large multi-model ensemble to investigate the robustness/uncertainty in the response of the atmosphere to El Niño Southern Oscillation (ENSO) in a present and a future climate. More precisely, we have used outputs of five climate models each run at least 100 times all starting with slightly different initial states of the atmosphere. These simulations are part of the international HAPPI project (also here). In this project, the future climate (+2ºC) is defined as a world in which the global mean temperature is higher by 1.2ºC relative to the present-day climate (or 2ºC higher than the pre-industrial climate of the 1800s). To reach this very mitigated warming target, the model is forced with SSTs that reflect their expected future state based on the multi-model mean from a CMIP5 experiment with increased greenhouse gases concentrations in the atmosphere.
The present-day typical response of the atmosphere to ENSO is characterised by a wave train propagating from the tropics towards North America and the North Atlantic. Over the North Pacific mid-latitudes, it translates in a deeper mean sea level pressure low than usual (grey contours in Fig. 1a) but there is a considerable member-to-member variability in the location (Fig. 1b) and amplitude (shading in Fig. 1a) of this negative anomaly. This spread is all due to the internal variability of the atmosphere.
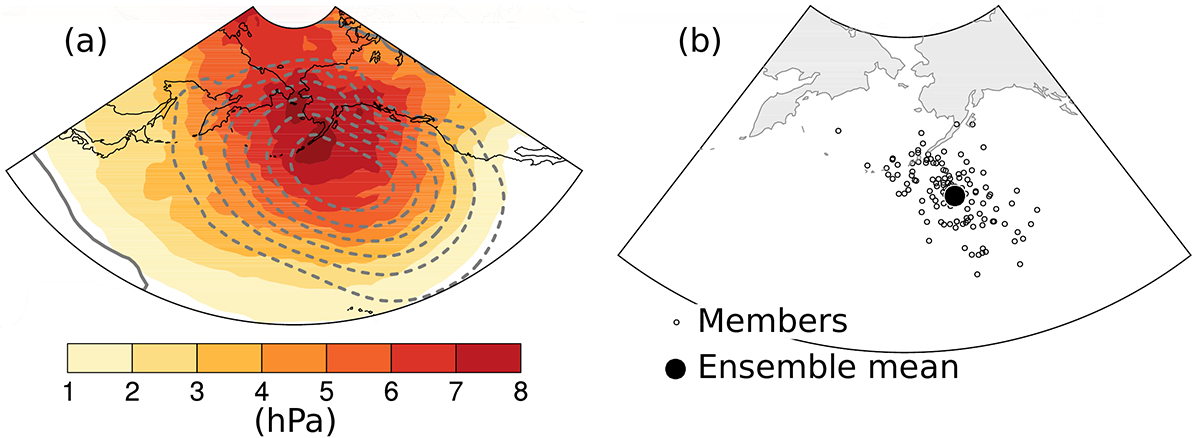
Under the weak 1.2ºC warming, the low over the North Pacific becomes deeper on average, especially on its northeastern flank (Fig. 2a). However, if only one random member of the present simulation is compared with one random member of the future simulation, there is a chance (1 chance out of 4 or 25% chance) of observing the opposite result, that is a pressure increase in the low (see the leftmost blue box in Fig. 2b). When more and more members are used, the response to the warming becomes more and more precise (or less and less uncertain), with the largest part of the distribution showing a deeper low (negative response). As an example, with the Norwegian model we need a minimum of 8 members to be relatively certain of seeing a deepening of the low on its northeastern flank (95% of 10,000 random combinations are negative) under a weak warming scenario (Fig. 2b). We expect that the larger the warming, the lower the minimum number of members required.
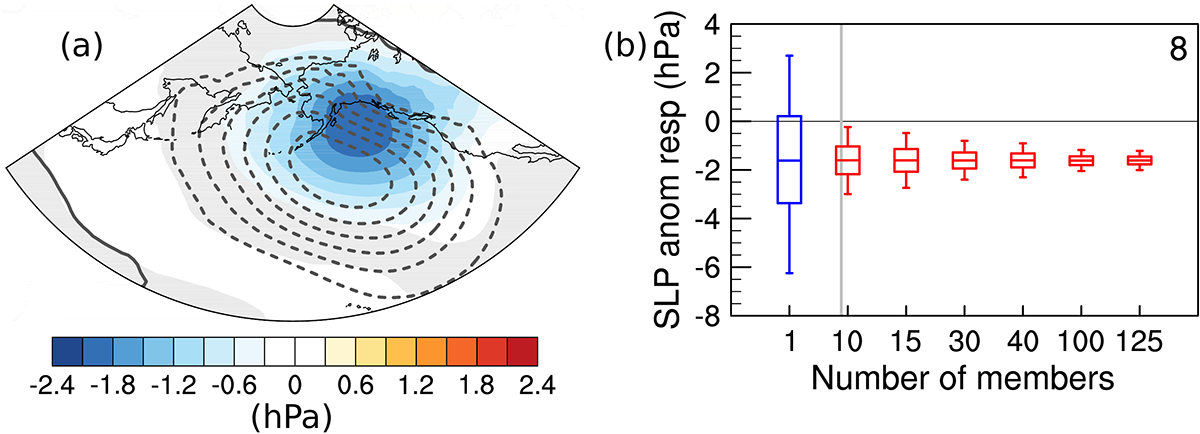
The wave train associated with ENSO modifies the atmospheric circulation at mid-latitudes, which affects weather over the whole Northern Hemisphere and more especially over North America, but the models may give different pictures. During El Niño, the warm phase of ENSO, Alaska experiences higher temperatures than usual in winter (Fig. 3a). However, although Alaska will still be warmer in the future, the change differs from model to model (Fig. 3b). In contrast, over Florida, a region wetter than usual in winter during El Niño (Fig. 3c), all the models agree on even more precipitation in the future (Fig. 3d). These changes are mainly due to the change in the mean state of the atmospheric circulation (Li et al. 2018) rather than to the increased atmospheric moisture in a warmer world.
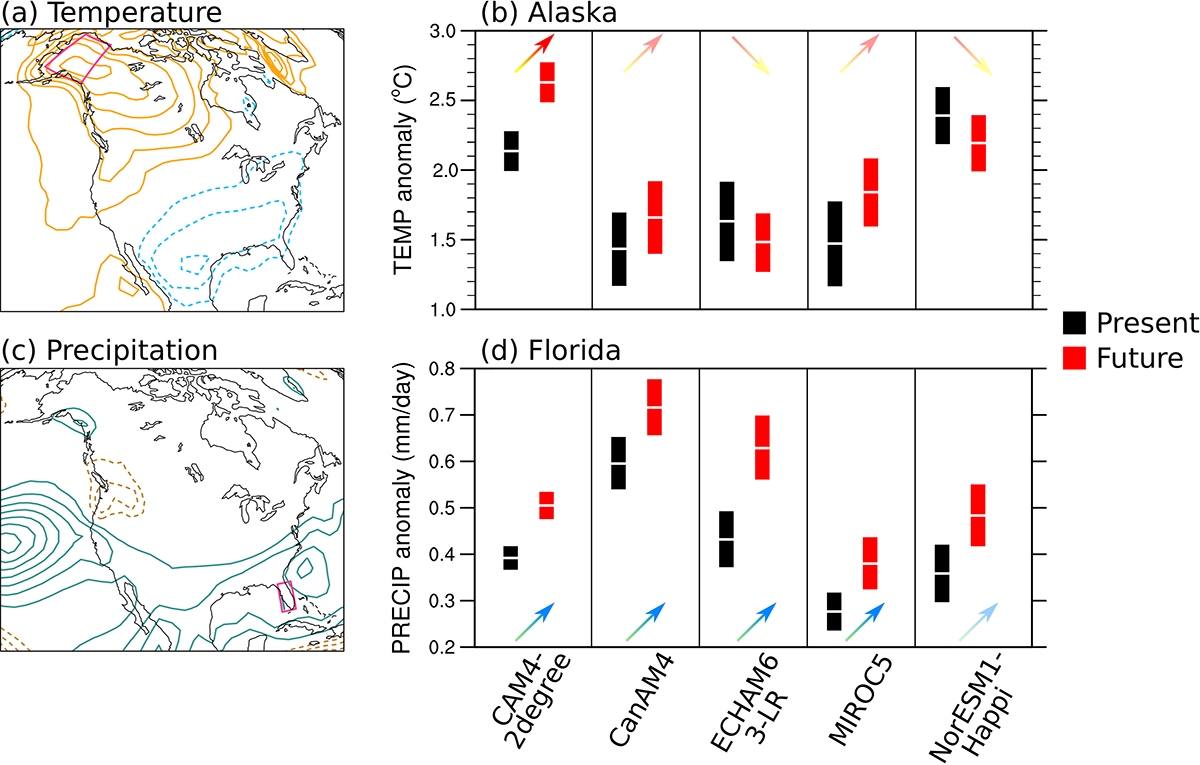
The responses to a weak global warming obtained in this study depend on several other factors that increase their uncertainty. Among them, several recent studies have pointed out biases in models that alter the SST response in the equatorial Pacific obtained within the CMIP5 framework on which the HAPPI experimental set-up is based. Moreover, the experiments are forced by the observed SSTs, which means that the ocean cannot react to atmospheric changes. To alleviate this issue, large ensembles of coupled models should be used. Finally, the observed response may be caused by multi-decadal variability of ENSO or the atmosphere and nonlinear sensitivity to the amount of warming.
To conclude, this study highlights the importance of using large enough ensembles of different models to precisely assess any change whether it be within the present period or in the future.
References
Deser, C., A. Phillips, V. Bourdette, and H. Teng, 2012: Uncertainty in climate change projections: the role of internal variability. Climate Dynamics, 38, 527-546, doi: 10.1007/s00382-010-0977-x
Li, C., and Coauthors, 2018: Midlatitude atmospheric circulation responses under 1.5 and 2.0ºC warming and implications for regional impacts. Earth System Dynamics, 9, 1-24, doi: 10.5194/esd-9-1-2018.
Lorenz, E. N., 1963: Deterministic nonperiodic flow. Journal of the Atmospheric Sciences, 20, 130-141, doi: 10.1175/1520-0469(1963)020<0130:DNF>2.0.CO;2.
Michel, C., C. Li, I. R. Simpson, I. Bethke, M. P. King, and S. Sobolowski, 2020: The change in the ENSO teleconnection under a low global warming scenario and the uncertainty due to internal variability. Journal of Climate, doi: 10.1175/JCLI-D-19-0730.1.
Washington, R., 2000: Quantifying chaos in the atmosphere. Progress in Physical Geography, 24, 499-514, doi: 10.1177/030913330002400402.