Written by Anne Gro Vea Salvanes and Elin Darelius from the Bjerknes Centre and the University of Bergen.
Loss of oxygen and expansion of oxygen depleted environments have been witnessed in both coastal and open-ocean systems since the middle of the 20th century, and ocean modelling predicts continuing decease by the year 2100. Oxygen depletion occurs thus during the same time epoch as global warming. Increased knowledge on how and why oxygen varies in space and time shapes the biogeochemical and ecological structure of marine systems and will be needed for future predictions of marine productivity. In coastal systems deoxygenation is also linked to human activities that lead to increased loadings of nutrients and organic matter, and to regional effects of climate induced changes in wind and precipitation patterns.
Masfjorden is one of the fjords showing recent oxygen depleted basin water and is part of a case study of the global review paper on “System controls of coastal and open ocean oxygen depletions” (Pitcher et al. 2021, Fig. 1) aiming to improve the understanding of deoxygenation and its impact using a global and multidisciplinary perspective.
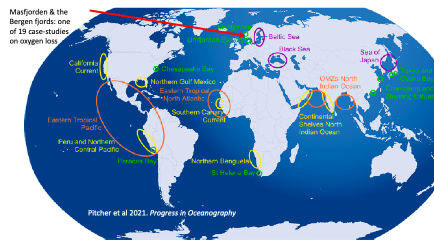
Oxygen data from the open ocean shows an average decrease in oceanic oxygen of about 2% since 1960, but estimates vary between ocean basins, regions, and water depth. Predictions from models indicate continued decrease of 1-7% by 2100, and additional calculations suggest that rates of deoxygenation are greater in coastal environments than in the open ocean. In 2008, the number of coastal sites reporting deoxygenation had increased by an order of magnitude compared to 1960. These trends led the Intergovernmental Oceanographic Committee (IOC) appoint the GO2NE expert group to provide a global multidisciplinary overview of the problem, which now is published as a review in Pitcher et al. (2021).
Sources and sinks of oxygen comes from many physical, biological and biogeochemical processes, and their imbalance can lead to deoxygenation. Photosynthesis and air-sea exchange drives oxygen concentrations in the surface waters. In bottom water of shallow areas exchange between the sediment-water interface is important. The bottom water of deeper basins such as deep fjord-basins of western Norway depend on horizontal and vertical transport (advection) from outside the fjords, and of that the water that enters is heavier than the basin water. Transport of oxygenated water across ecosystem boundaries and mixing and diffusion are important physical processes in determining the vertical distribution of oxygen.
Production of oxygen occurs by photosynthesis and the production of phytoplankton and organic matter in the surface waters (euphotic zone) and oxygen consumption occur through respiration and remineralization in the entire water column. Fish, invertebrates, and all marine organisms require oxygen to live and grow, and microorganisms such as bacteria and virus consume oxygen when they disintegrate organic matter (feces from fish, pellets from zooplankton, dead phytoplankton, and other organisms) as it sinks down the water column. If there is strong stratification this limits vertical mixing, and which lead to long residence times of deep-water masses isolated from oxygen supply.
The Pitcher et al. (2021) paper provides a review of system-specific drivers of low oxygen in a range of case studies, including west Norwegian fjords, continental shelves, enclosed seas, coastal environment, and the open ocean. Each case study describe the system, including present oxygen conditions and known trends of oxygen concentrations over time. In the open ocean the water masses depletion of oxygen has led to development of major subsurface oxygen minimum zones (OMZ) between 100 and 1000 m depth in the eastern part of the tropical Pacific and Atlantic oceans and in the northern Indian ocean. OMZ’s reach lowest oxygen concentrations in subtropical and tropical upwelling regions (EBUEs) such as the Benguela and the California upwelling ecosystems. Shelf systems and their oxygen concentration are influenced by the adjacent coastal zone and the open ocean.
On the coast through freshwater supply and river-borne nutrient loadings, and the origin of water masses advected onto the shelves. Continental shelves of EBUE’s are characterized by low oxygen concentrations generated by high productivity without any direct anthropogenic influence. Enclosed seas such as the Baltic Sea and the Black Sea are connected to the open ocean at the surface by one or more narrow straits bounded at depth by a submarine ridge. Both show records of naturally oxygen depletion the last 8000 years, but increased nutrient supply has strengthened the negative developments, and for example the Baltic Sea is now considered the largest “human induced” hypoxic area in the world (Pitcher et al. 2021).
The Coastal zone and environments here show considerable spatial complexity with respect to oxygen content. Those with restricted circulation and long water residence times appears to have low oxygen concentrations, and if the inorganic nutrient supply increases due to natural or cultural eutrophication, these environments will have increased susceptibility to hypoxia. Here restricted circulation serves both to limit ventilation as well as to aid in accumulating organic matter to be degraded in bottom waters. Fjords represent such environments. The oxygen contents of the basin waters of the west Norwegian fjords are largely dependent on basin water renewal frequency, which in turn depend on fjord topography (sill-depth, the cross-sectional area at the sill, and the basin depth below the sill) as well as of the density of the water masses outside the fjord (Aksnes et al. 2019). The focus in the following is mainly on Masfjorden and the Bergen fjords.
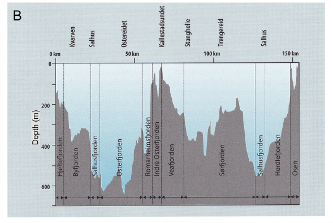
From the comprehensive study of Gaarder (1916), reporting studies of the oceanography of west Norwegian fjords 105-115 years ago, it is evident that some fjords are naturally and permanent hypoxic, and others temporary hypoxic. Mofjorden (max depth 200 m, 700 m long sill ca 2.5 m deep) in the inner part of Osterfjorden, and Nordåsvannet (max depth 80 m, 30-40 m long and 3 m deep sill) are two fjords known for hypoxic and sulphidic waters.
Presence of oxygen depleted basin water is mainly due to the fjord topography and the presence of shallow sills at the entrance of fjords and their distance to well oxygenated water masses on the coast. Above the sea surface we can see mountains and valleys varying in depth and with. But that is exactly how the topography looks under the sea as well in the Bergen fjord area (Fig. 2). This means that any fjord basin has a sill that is shallower than its basin, and this influence renewal of oxygen in the basin water.
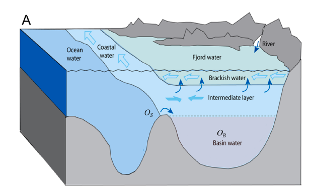
Three major water masses are present in the fjords. The surface layer is a brackish layer formed by fresh water supply from the local watershed, the intermediate layer is between the brackish layer and the sill depth and has largely the same properties as at similar depths outside the fjord (Aksnes et al. 2019, Fig. 3). The Norwegian Coastal Current (Fig. 4) and the wind patterns along the Norwegian coast affect the circulation of the intermediate layer (Aksnes et al. 2019). The basin water is located between the sill depth and the maximum fjord depth (Fig. 3).
The three main processes that control the basin water oxygen concentration are i) oxygen consumption due to biological activity; ii) vertical turbulent diffusion; and iii) basin water renewals caused by intrusion of water with density that exceeds the density of existing basin water (i.e. the water that enters must be heavier than the “old” basin water) (Aure and Stigebrandt 1989; Aksnes et al. 2019).
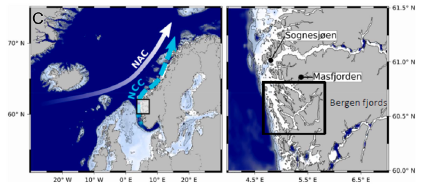
In Masfjorden, it was observed a decreasing trend (0.047 ml l-1 yr-1) of oxygen concentration and basin water density in the basin water in the period 1975-2017 and a 1 oC increase in temperature (Aksnes et al, Fig. 5). There was a clear decreasing oxygen concentration from ca 200 m depth to the seabed that appears to have been initiated around 2014 and which showed the lowest oxygen level in 2017 when the basin water was hypoxic (< 2 ml/l dissolved oxygen) from ca 300 m and to the seabed at ca 500 m depth (Pitcher et al. 2021, Fig. 6). Oxygen levels of the basin water increased slightly the following years, though by the early spring of 2021 when the Geophysical Institute and the HypOnFjordFish had fieldwork in the fjord, Masfjorden was still not considered as having had any major inflow of heavier and oxygen rich water from outside the fjord.
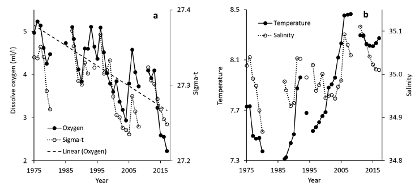
So, what do we know about when the typical timing of renewal of basin waters of west Norwegian fjords occur? Data from Byfjorden and the Bergen fjords were scrutinized by Sætre et al. (2010 and shown on Fig. 7) and found to match in time when there is typically strong winds from north along the Norwegian coast. This appears to happen between April and June/July, and so in the spring/early summer. Northern winds lead to the Norwegian Coastal water becoming shallow and broad and allowing the deeper and heavier water being transported/advected over the fjord sills (Sætre 2007). Mean annual variation (1981-2004) in oxygen and temperature at 300 m shows that oxygen levels increase, and temperature decrease during a typical spring/early summer renewal period (Sætre et al. 2010; Fig. 5.3.2.a of Pitcher et al 2021, Fig. 7).
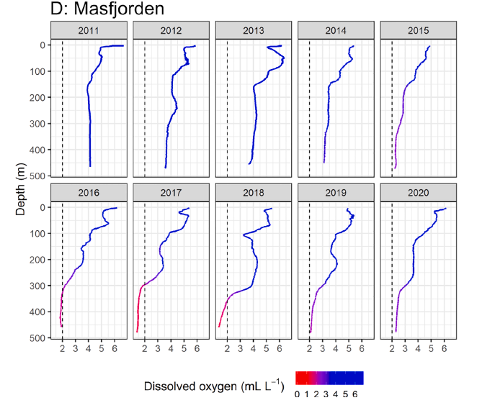
Findings reported in Aksnes et al. (2019) and Darelius suggest that it is more than ten years ago since the last deep-water renewal in Masfjorden. This year, it appears, however, to have been some inflow of better oxygenated waters into Masfjorden in spring/early summer. Lars Asplin, our colleague at the Institute of Marine Research, took CTD-casts in Masfjorden in April and mid-August of 2021 and sent us an urgent message that it appears Masfjorden now has received some new basin water: have a look in the blog: https://elindarelius.no/category/fjord-oceanography/ .
These are great news for the organisms that live at great depths of Masfjorden, and indeed for the HypOnFjordFish project that now will have data and samples of mesopelagic and other fish and marine organisms from before, during and after a hypoxic period. It is only two weeks until the next biological research cruise with Master students to Masfjorden and Fensfjorden.
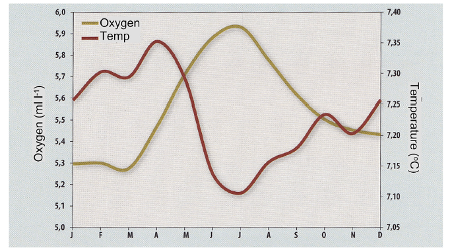
Fjord researchers in Bergen also got great news this summer – as two multi-disciplinary projects focusing on fjords and hypoxia got funded. In the years to come, colleagues from Bjerknes will within the framework of FJO2RD (RCN) and CLIFORD (BCCR, internal funding) start up activity centered around Masfjorden and Lurefjorden. Sediment cores will reveal the oxygenation history and related biodiversity changes in the recent past; oceanographic moorings, historical data and modelling efforts will tell us about the renewal process itself and the connection between the fjords, the coast and the open ocean whereas the biologists will study the effect of changing oxygen conditions on the marine life in the fjords.
References
Aksnes, D.L., Aure, J., Johansen, P.-O., Johnsen, G.H., Salvanes, A.G.V., 2019. Multi-decadal warming of Atlantic water and associated decline of dissolved oxygen in a deep fjord. Estuarine Coastal and Shelf Science 228, 106392, https://doi.org/10.1016/j.ecss.2019.106392
Aure, J., Stigebrandt, A., 1989. On the influence of topographic factors upon the oxygen consumption rate in sill basins of fjords. Estuarine Coastal and Shelf Science 28, 59–69.
Darelius, E., 2020. On the effect of climate change on deep water renewal frequency in sill fjords. Estuarine Coastal and Shelf Science. https://doi.org/10.1016/j.ecss.2020.106904
Gaarder, T., 1916. De vestlandske fjorders hydrografi (The hydrography of the west Norwegian fjords, in Norwegian). Bergen Museums Aarbok 1915-1916. Naturvidensk. Række. Nr. 2, 200 pp.
Grant C. Pitcher, Arturo Aguirre-Velarde, Denise Breitburg, Jorge Cardich, Jacob Carstensen, Daniel J. Conley, Boris Dewitte, Anja Engel, Dante Espinoza Morriberón, Georgina Flores, Véronique Garçon, Michelle Graco, Marilaure Grégoire, Dimitri Gutiérrez, José Martin Hernandez-Ayon, Huai-Hsuan May Huang, Kirsten Isensee, María Elena Jacinto, Lisa Levin, Alberto Lorenzo, Eric Machu, Lander Merma, Ivonne Montes, Naqvi SWA, Aurelien Paulmier, Michael Roman, Kenneth Rose, Raleigh Hood, Nancy N. Rabalais, Anne Gro Vea Salvanes, Renato Salvatteci, SoniaSánchez, Abdelfettah Sifeddine, Abdoul Wahab Tall, Anja K. van der Plas, Moriaki Yasuhara, Jing Zhang, ZY Zhu (2021). System controls of coastal and open ocean oxygen depletion. Progress in Oceanography. https://doi.org/10.1016/j.pocean.2021.102613
Sætre, R (editor), The Norwegian Coastal Current, 2007. Tapir Academic press
Sætre, R., Aure, J., Gade, H.G., 2010. Oseanografi og klima. In: Johannessen, P., Sætre, R., Kryvi, H., Hjelle, H. (Eds.), Bergensfjordene - Natur Og Bruk. Havforskningsinstituttet, Uni Research, Universitetet i Bergen, Bergen Kommune, John Grieg AS, Bergen, pp. 33–55.